ATMOS
Atmospheric and Climate Science Lab.

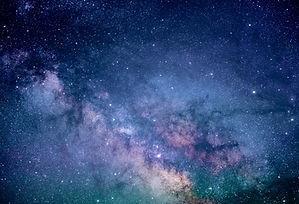
Water Vapour and Climate Change
SIGNIFICANCE
Water vapour and its role in typical Earth water cycle
The atmosphere is constantly replenished with water. Water vaporises near the surface of the earth and is carried upward by warm air currents. It condenses into clouds, travels with the wind, and eventually returns to Earth as rain or snow. The movement of heat and energy from the surface of the Earth to the atmosphere and its subsequent distribution across the planet occurs in part through this cycle

(Earth system model of water cycle, where water vapour plays a major role. Source: https://ugc.berkeley.edu/background-content/water-cycle/)
Water vapour is the phase of water that exists in the gaseous state. It is one of the water states in the hydrosphere. The evaporation or boiling of liquid water or the sublimation of ice can produce water vapour. Similar to other atmospheric components, water vapour is transparent. Under ordinary atmospheric circumstances, water vapour is continuously produced and dissipated by evaporation and condensation, respectively. It is less dense than most other air components and causes convection currents that can lead to cloud formation
Measurement of atmospheric water vapour
Various techniques and observational platforms can be used to measure water vapour in the atmosphere. These observations are utilised mostly for numerical weather forecasting, monitoring, and climate and atmospheric chemistry research. In-situ measurements of water vapour are performed by balloon and aircraft-borne equipment, as well as remotely by satellite and ground-based sensors.
The following are some examples of the several methods that can be used to measure water vapour:
Polar-orbiting platforms with passive microwave sensors.
Infrared sensors, which have been used by satellites for the longest time to measure and profile water vapour.
Ultraviolet, visible, and near-infrared imagers are retrieval methods that are used throughout the daytime. These imagers use two channels and provide a high level of spatial resolution.
Limb sounding is a method that involves sounding to the different levels of the atmosphere by making observations along a tangent ray that does not pass through the surface of the earth.
Radiosondes are a type of instrument that is frequently utilised for in-situ sounding and are known for the high-quality profiles of relative humidity and vapour pressure that they produce.
Frost point hygrometers that are carried aloft by balloons and use a cooled mirror, the temperature of which is carefully maintained at the frost point temperature.
Equipment situated on the ground, which are capable of conducting semi-continuous probing of the air mass over a specific place.
A variety of long-range commercial aircraft fitted with vapour sensors.
Challenges in observing water vapour
Due to a lack of observations, our understanding of water vapour distribution in the upper troposphere and stratosphere is limited. Very little is known about the global distribution of water vapour in the upper troposphere and the stratosphere because of the scarcity of high vertical resolution observations in these layers of the atmosphere. Data from satellites, frost point hygrometers, and meteorological reanalyses don't always agree with one another. We require more precise data with expanded geographic coverage. We don't have a good grasp on how water vapour ascends into the stratosphere, and that's reflected in our inability to make sense of the observed temporal trends in water vapour there.
Precipitable water vapour
Precipitable water vapour (PWV) is the total amount of atmospheric water vapour that would be found in a vertical column with a unit-cross sectional area if the water vapour were to be completely condensed into a liquid and collected in that same unit volume. This definition describes the amount of water vapour that would be precipitated if the water vapour were to fall as precipitation. This value is typically stated in terms of height, using either metres or centimetres as the unit of measurement. To put it another way, it is a measurement of the amount of water that can be collected from the top of the atmosphere to the surface at any given time through the process of condensation.
Importance of water vapour in climate science
The most abundant greenhouse gas known as water vapour. It is responsible for approximately half of the warming that is occurring on the planet. As is the case with other greenhouse gases, it allows practically all of the sunlight to reach the surface of the earth, but it absorbs the heat that is emitted upward from the surface.
The majority of us are familiar with water vapour role as a greenhouse gas. When there is a high amount of humidity in the air, the air temperature drops gradually as the sun goes down. This is because there are high volumes of water vapour in the atmosphere, which traps heat. On the other hand, temperatures decrease significantly during the night in dry climates due to the low levels of heat-trapping water vapour that are present in the atmosphere. When you move from the bright sunlight into the darker shadows, you will feel the same way. If there is a lot of humidity, you won't notice much of a difference in temperature, but if there isn't much humidity, you'll notice a bigger difference.

(Role of water vapour in climate science, indicating different processes. Source: Gerard et al., 2004
Temperature has a significant impact on the amount of water vapour that an atmosphere can store. When temperatures are extremely low, it has a maximum holding capacity of only 0.01%, but when temps are approximately 90 degrees, it has a maximum holding capacity of 3%. Because of this, when the earth heats, the atmosphere is able to contain a greater amount of water vapour. To be more specific, the atmosphere is capable of holding around 7% extra water vapour for every one degree Celsius that is added to the temperature (Trenberth et al., 2005).
Water vapour also plays an important role in weather formation. For instance, it releases latent heat through condensation and precipitation processes. The condensation process causes the precipitation, which fall down on the earths surface in different forms.
Water vapour-temperature feedback mechanism
The amount of water vapour in the atmosphere cannot freely vary, which is not the case for the concentrations of other greenhouse gases. In its place, it is determined by the temperature of the lower atmosphere and the surface through the application of a physical relationship known as the Clausius-Clapeyron equation. This equation is named after the German physicist Rudolf Clausius and the French engineer Émile Clapeyron, both of whom lived in the 19th century.
This relationship suggests that an increase in the capacity of air to hold water vapour is a function of an increase in the temperature of that volume of air, assuming that there is a liquid water surface in equilibrium with the atmosphere. This is based on the assumption that there is a liquid water surface. This assumption holds true to a certain extent over the oceans, which contain copious amounts of water, but it is not valid across the continents. Because of this, the relative humidity, which is defined as the amount of water vapour present in the air in relation to its capacity, is close to one hundred percent over ocean regions, whereas it is significantly lower over continental regions (approaching 0 percent in arid regions).
It should not come as a surprise that the average relative humidity of the lower atmosphere of Earth is comparable to the portion of the planet's surface that is covered by seas (that is, roughly 70 percent). It is anticipated that this value will stay relatively stable regardless of whether the Earth warms up or cools down. The modification of land by humans, such as the clearing of tropical forests and the introduction of irrigation systems, can have an impact on the relative humidity of large swaths of land and even on a regional scale. This could result in slight shifts in the global relative humidity.
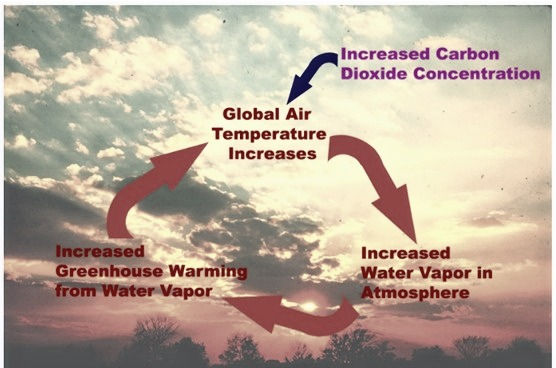
(Mechanism behind a positive water vapour feedback on temperature. Source: NASA climate change
When the temperature of the atmosphere rises, there will be a corresponding increase in the amount of water vapour that is present in the atmosphere. The net greenhouse effect actually increases stronger as the surface warms, which leads to even greater warming as a result of the feedback loop. This is due to the fact that water vapour is an extremely potent greenhouse gas, even more potent than CO2. The name given to this type of positive feedback is the "water vapour feedback." It is the fundamental reason why climate sensitivity is significantly higher than the previously stated theoretical value of 0.25 degrees Celsius (0.45 degrees Fahrenheit) for each increase of 1 watt per square metre of radiative forcing
Water vapour as a most abundant greenhouse gas
“Carbon Dioxide Versus Water Vapour: Who "Wins" In Climate Warming?”
Water vapor is also the most important greenhouse gas in the atmosphere. Heat radiated from Earth's surface is absorbed by water vapor molecules in the lower atmosphere. The water vapor molecules, in turn, radiate heat in all directions. Some of the heat returns to the Earth's surface. Thus, water vapor is a second source of warmth (in addition to sunlight) at the Earth's surface.
Although it is well established that water vapour is the most abundant greenhouse gas on Earth, the degree to which it contributes to overall temperature increases has been the topic of considerable debate. Researchers have validated the function of the gas as a crucial component of climate change by estimating the heat-trapping effect of water in the air with greater precision than they have ever been able to do before using current satellite data from NASA.
The heat-amplifying effect of water vapour was validated by Andrew Dessler and colleagues from Texas A&M University in College Station. They found that the effect is powerful enough to twice the climatic warming that is induced by higher amounts of carbon dioxide in the atmosphere.
Current status of global water vapour
According to Allan et al. (2022), the amount of water vapour in the atmosphere has been rising steadily since 1979. Observations made by microwave satellites showed that the global mean column integrated water vapour increased by 1% each decade between 1988 and 2014. When combined with the observed global surface warming trend of 0.17 K/decade, this amounts to global moisture increases with warming of approximately 6%/K, which is near to, but slightly lower than, that which is anticipated by the Clausius Clapeyron equation.
They also revealed that the substantial increases in upper tropospheric water vapour over time are bigger than the changes in moisture content that occur at lower tropospheric levels at a percentage per decade of approximately lower tropospheric levels. On the basis of the Clausius-Clapeyron temperature dependence of moisture response to warming and enhanced air warming aloft due to radiative convective balance, this is a well understood phenomenon. This is especially true over tropical oceans (Held & Soden, 2006; Ohmura, 2012). This is consistent with minor changes in the relative humidity of the upper troposphere that have been derived from satellite measurements, which confirms previous findings (e.g., Soden et al., 2005).
In addition, throughout the course of interannual timescales, the average near-surface water vapour concentration over the world rises by around 5% for every K that the surface temperature rises. A greater sensitivity of 10%–15% increase in 300 hPa water vapour per K increase in surface temperature is primarily related to changes over the tropical ocean and is explained by thermodynamic amplification of upper tropospheric temperature changes and the Clausius Clapeyron temperature dependence of saturation vapour pressure. This larger sensitivity is primarily related to changes over the tropical ocean (Dessler et al., 2008; Held & Soden, 2006).
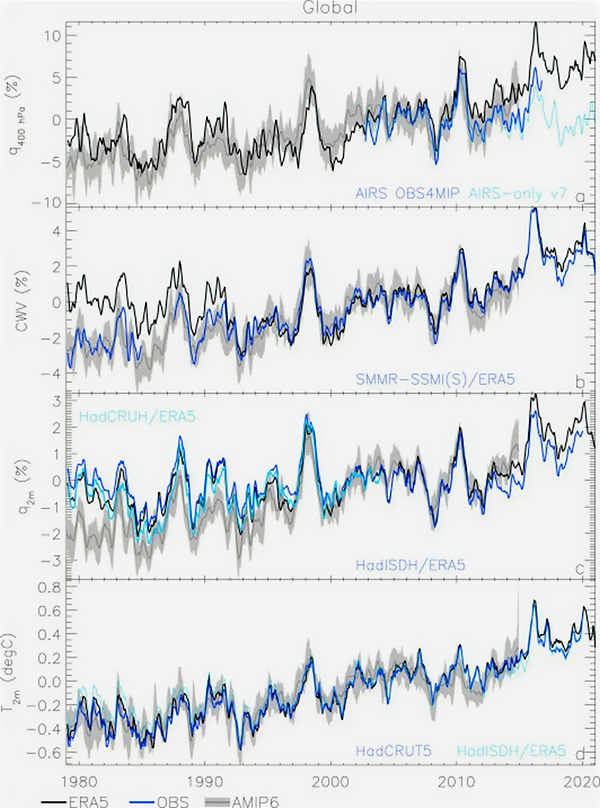
(Anomaly in water vapour, specific humidity and temperature in the troposphere for the period 1979-2020: Allan et al., 2022)
Website for data:
Satellite Measurements:
AIRS and MLS water vapour data:
https://disc.gsfc.nasa.gov/datasets?keywords=AIRS%20water%20vapor&page=1
MODIS data:
https://disc.gsfc.nasa.gov/datasets?keywords=MODIS%20water%20vapor&page=1
GOME total column water vapour product:
https://earth.esa.int/eogateway/catalog/gome-total-column-water-vapour-climate-product
SSM/I
https://www.remss.com/missions/ssmi/
SCHIMACHY:
https://www.sciamachy.org/products/index.php?species=H2O
INSAT 3D data:
SHWOOH data:
https://csl.noaa.gov/groups/csl8/swoosh/
GOZCARD data:
https://disc.gsfc.nasa.gov/datasets/GozMmlpH2O_1/summary
SPARC data:
https://www.sparc-climate.org/activities/previous-activities/water-vapour/
Reanalysis Data:
ERA 5:
https://cds.climate.copernicus.eu/#!/search?text=ERA5&type=dataset
MERRA2:
https://disc.gsfc.nasa.gov/datasets?keywords=MERRA2%20water%20vapour&page=1
NCEP/NCAR:
https://developers.google.com/earth-engine/datasets/catalog/NCEP_RE_surface_wv
JRA55:
https://climatedataguide.ucar.edu/climate-data/jra-55
CAMS:
https://ads.atmosphere.copernicus.eu/#!/home
Ground based Observation:
Radiosonde:
IGRA_v02: https://www.ncei.noaa.gov/access/metadata/landing-page/bin/iso?id=gov.noaa.ncdc:C00975
GNSS data:
GPS derived precipitable water vapour:
https://www.mosdac.gov.in/gps-derived-integrated-water-vapour
Ground based Stratospheric water vapour data
https://gml.noaa.gov/ozwv/wvap/
Future Projected CMIP6 data:
CDS:
https://cds.climate.copernicus.eu/cdsapp#!/dataset/projections-cmip6?tab=overview
WCRP:
https://www.wcrp-climate.org/wgcm-cmip/wgcm-cmip6
Important Paper:
-
Dai, A. 2006. Recent Climatology, Variability, and Trends in Global Surface Humidity. J. Climate. 19, 3589–3606. https://doi.org/10.1175/JCLI3816.1
-
Held, I. M. and Soden, B. J. 2000. Water vapour feedback and global warming. Ann Rev Energy Environ. 25, 441–475.
-
Ross, R. J. and Elliott, W. P. 1996. Tropospheric water vapour climatology and trends over North America. J Climate. 9, 3561– 3574.
-
Ross, R. J. and Elliott, W. P. 2001. Radiosonde-based Northern Hemisphere tropospheric water vapour trends. J Climate. 14, 1602–1611.
-
Trenberth, K.E., Fasullo, J., Smith, L., 2005. Trends and variability in column-integrated atmospheric water vapour. Climate Dynamics. 24, 741–758. https://doi.org/10.1007/s00382-005-0017-4
-
Trenberth, K. E., Fasullo, J. T., Kiehl, J., 2009. Earth’s global energy budget. Bull. Amer. Meteor. Soc. 90, 311–324. doi:10.1175/ 2008BAMS2634.1
-
Zhang, L., Wu, L., Gan B., 2013. Modes and mechanisms of global water vapour variability over the twentieth century. J. Clim. 26(15), 5578–5593. doi:10.1175/JCLI-D-12-00585.1
-
Susan Solomon, Karen H. Rosenlof, Robert W. Portmann, John S. Daniel, Sean M. Davis, Todd J. Sanford, Gian-Kasper Plattner, Contributions of Stratospheric Water Vapor to Decadal Changes in the Rate of Global Warming, Science, 10.1126/science.1182488, 327, 5970, (1219-1223), (2010).
-
Dessler, A. E., Schoeberl, M. R., Wang, T., Davis, S. M., and Rosenlof, K. H.: Stratospheric water vapor feedback, P. Natl. Acad. Sci. USA, 110, 18087–18091, https://doi.org/10.1073/pnas.1310344110, 2013.
-
Hegglin, M., Plummer, D., Shepherd, T. et al. Vertical structure of stratospheric water vapour trends derived from merged satellite data. Nature Geosci 7, 768–776 (2014). https://doi.org/10.1038/ngeo2236